Share
Tweet
Share
Share
Abstract: Hybrid Electric Vehicles (HEVs) represent a transformative advancement in the automotive industry, combining internal combustion engines (ICE) with electric propulsion systems to achieve superior fuel efficiency and reduced emissions. This paper explores the core components and systems integral to HEV functionality, including propulsion system design, advanced control strategies, battery technologies, and thermal management solutions. The design section examines the synergy between ICE and electric motors, managed by sophisticated power electronics controllers, to optimize energy utilization. Control systems are categorized into rule-based and optimization-based approaches, highlighting their role in seamless energy flow and adaptive performance across diverse driving conditions. The paper delves into battery technologies, comparing nickel-metal hydride (NiMH) and lithium-ion (Li-ion) systems, emphasizing energy density, lifecycle advantages, and the critical function of battery management systems (BMS) in ensuring reliability and safety. Furthermore, thermal management innovations, such as refrigerant-based cooling systems and high-voltage coolant heaters, are analyzed for their contributions to operational efficiency and longevity. The environmental and economic impacts of HEVs are also discussed, emphasizing their potential to mitigate greenhouse gas emissions and dependence on fossil fuels. Challenges such as infrastructure gaps, integration complexities, and public adoption barriers are addressed alongside future trends in electrification and hybrid system advancements. This comprehensive review underscores the importance of multidisciplinary innovation in overcoming the challenges and enhancing the sustainability of HEVs.
Hybrid electric vehicles (HEVs) are an innovative solution in the automotive industry, integrating both conventional internal combustion engines (ICE) and electric propulsion systems to enhance fuel efficiency and reduce emissions[1][2]. These vehicles are designed with a dual powertrain system, combining the use of fossil fuels and electric energy, which positions them as a sustainable alternative to traditional vehicles[3]. The design of HEVs is centered around several critical components, including the internal combustion engine, electric motors, and a sophisticated power electronics controller, all contributing to the vehicle’s operational efficiency[1][4].
Control systems in HEVs are pivotal in managing the dynamic interplay between various vehicle components, ensuring seamless operation across diverse driving conditions[5]. These systems are divided into rule-based and optimization-based categories, each offering distinct strategies for vehicle performance management[5]. The Power Electronics Controller plays a vital role, regulating energy flow from the traction battery and managing the electric traction motor’s speed and torque, thus maintaining smooth transitions between different modes of operation[1][6]. Energy management strategies, including advanced cooling methods, are crucial for optimizing battery life and vehicle performance[7].
The battery systems in HEVs, predominantly nickel-metal hydride (NiMH) and lithium-ion (Li-ion) batteries, are integral to vehicle performance and efficiency[8][9]. These batteries provide the necessary energy storage capabilities, with Li-ion batteries offering advantages in terms of energy density and lifecycle[10]. Battery management systems (BMS) ensure the safe and reliable operation of these energy sources, overseeing charge control, cell balancing, and thermal management to maintain optimal performance across varying conditions[11].
Thermal management and cooling systems are essential in maintaining the operational efficiency of HEVs, ensuring both batteries and power electronics function within safe temperature ranges[12][13]. These systems utilize advanced technologies, such as refrigerant-based circuits and high-voltage coolant heaters, to manage temperature effectively, contributing to the overall longevity and efficiency of the vehicle[12]. As HEVs continue to evolve, advancements in cooling system design and energy management strategies will be pivotal in addressing the challenges and enhancing the sustainability of these vehicles[14].
Design of Hybrid Electric Vehicles
Hybrid electric vehicles (HEVs) are designed to integrate two primary types of propulsion systems: a conventional internal combustion engine (ICE) and one or more electric motors powered by batteries[1][3]. This dual system aims to enhance fuel efficiency and reduce emissions by optimally combining the use of fossil fuels and electric energy[2].
The design of HEVs includes several critical components. The internal combustion engine operates using a spark-ignited system, where fuel is injected into either the intake manifold or the combustion chamber and ignited by a spark plug[1]. Complementing this, the electric motors are powered by energy stored in batteries, which are charged through regenerative braking and by the ICE itself[1][3]. Unlike purely electric vehicles, HEVs cannot be plugged in to charge their batteries[1].
A key feature of HEVs is the power electronics controller, which manages the flow of electrical energy delivered by the traction battery. This controller regulates the speed of the electric traction motor and the torque it produces, ensuring efficient power delivery[1]. The thermal system is another vital component, maintaining optimal operating temperatures for the engine, electric motor, and power electronics[1][4].
Moreover, HEVs typically employ an electronic continuously variable transmission (eCVT). This system contributes to improved fuel economy but can sometimes produce a monotone droning sound from the gasoline engine[15]. The inclusion of such advanced transmission systems underscores the emphasis on smooth driving and fuel efficiency in HEV design[15].
Control systems in HEVs are sophisticated, often classified into rule-based and optimization-based systems. These systems help manage the vehicle’s performance by adapting to varying driving conditions[5]. Additionally, evolutionary algorithms are being researched to further enhance the efficiency of HEVs by optimally combining gasoline and electric power based on the driving route[2].
Control Systems
Control systems in hybrid electric vehicles (HEVs) are crucial for managing the complex interplay between the various components of the vehicle. These systems can be categorized into two main types: rule-based and optimization-based control systems[5]. Rule-based control systems follow predefined rules and strategies for managing the vehicle’s operations, while optimization-based systems focus on achieving optimal performance through continuous adjustments and learning[5].
One key function of these control systems is onboard diagnostics (OBD), which is relatively low in cost but significantly beneficial for vehicle maintenance and operation[16]. The complexity of HEV control systems is highlighted by the numerous operational modes and the integration of diverse components[16].
The Power Electronics Controller plays a critical role within the control system, as it manages the flow of electrical energy from the traction battery, thereby regulating the speed and torque of the electric traction motor[1]. This is essential for maintaining efficient vehicle performance and ensuring smooth transitions between different driving conditions.
Furthermore, energy management strategies within the control system can substantially lower overall energy consumption. For instance, employing a distributed forced convection cooling method can maintain battery temperature within optimal limits, which is crucial for enhancing battery life and performance[7]. Additionally, innovative configurations like clutching the AC compressor motor during braking intervals help reduce energy use and vehicle weight[7].
The Engine Control Unit (ECU) is another pivotal component within the Powertrain Control Module (PCM) that ensures the engine operates at optimal efficiency[6]. This highlights the intricate nature of control systems in HEVs, which serve as the ‘brain’ of the vehicle, orchestrating the functions of its various subsystems[6].
Battery Systems
Hybrid electric vehicles (HEVs) utilize various battery systems to enhance performance and efficiency. The most commonly used batteries in HEVs are nickel-metal hydride (NiMH) and lithium-ion (Li-ion) batteries. NiMH batteries are renowned for their high-energy density and excellent power-to-weight ratio, making them suitable for the energy storage requirements of hybrid vehicles[8]. They are particularly favored for their robustness and reliability across diverse temperature conditions, which is essential for the varied charging and discharging cycles typical in hybrid driving scenarios[10].
Li-ion batteries, on the other hand, are lighter and have a higher energy density than lead-acid batteries, allowing hybrid vehicles to achieve longer ranges and improved performance. They offer advantages in terms of energy and power density, providing better high-temperature performance, longer life, and lower self-discharge rates[8][9]. Li-ion batteries have become the cornerstone of electric vehicle propulsion systems due to their ability to cater to different performance needs. They are available in various designs, including Lithium-Nickel Cobalt Aluminum Oxide (NCA), Nickel Manganese Cobalt Oxide (NMC), and Lithium Iron Phosphate (LFP), each with different balances of cost, power output, and energy density[10].
Battery management systems (BMS) are critical components in both electric and hybrid electric vehicles. They ensure the safe and reliable operation of the battery by implementing state monitoring and evaluation, charge control, and cell balancing. These systems manage the charge time, discharge strategy, cell equalization, and thermal management among the cells. The real-time data collected is essential for maintaining system safety and communicating battery status to the user interface[11]. As a complex electrochemical product, batteries in hybrid vehicles require precise management to handle uncertainties in performance across various operational and environmental conditions[11].
Furthermore, sophisticated thermal management systems are essential to maintain optimal temperature ranges for the battery, which can significantly impact its efficiency and lifespan. These systems typically involve a refrigerant-based circuit that includes components like condensers and evaporators to regulate the temperature of battery units under varying driving and ambient conditions[12][13]. This ensures that hybrid vehicles can operate with maximum efficiency and safety.
Thermal Management and Cooling
Thermal management and cooling are critical components in the design and operation of hybrid electric vehicles (HEVs). These systems ensure that both the batteries and power electronics are maintained within optimal temperature ranges, thereby enhancing performance and longevity.
Battery Cooling Systems
Battery cooling systems in hybrid electric vehicles are designed to maintain the ideal operating temperature, generally between 15 °C and 30 °C[12]. This is achieved through a secondary circuit that integrates coolant, composed of water and glycol, flowing through a cooling plate within the battery block. This circuit can transfer heat to a refrigerant from the vehicle’s air-conditioning system in a compact space, utilizing a high power density heat exchanger[12]. In situations where indirect battery cooling is insufficient, the coolant can be additionally cooled via a chiller, a special heat exchanger integrated into both refrigerant and coolant circuits[12]. These systems are designed to be compact and lightweight, making them suitable for the limited space in electric vehicles, thus not adding unnecessary weight or bulk[17].
Power Electronics and Charger Cooling
Cooling systems are also essential for maintaining the optimal operating temperature of power electronics and charger modules in HEVs. These systems often employ a liquid coolant that absorbs heat generated during operation, ensuring that the electronics remain within safe conditions[13]. The integration of air-conditioning components is increasingly important, as they directly or indirectly influence the cooling of batteries and electronics[4].
Advanced Thermal Management Features
Some hybrid electric vehicles are equipped with high-voltage coolant heaters that provide adequate temperature control at low outside temperatures, ensuring efficient battery operation[12]. Additionally, thermal systems are used to cool both the power electronics and the charger modules, which are crucial due to the heat generated by the electric motors under different operating conditions[13][18]. Smartphone apps have emerged as a modern feature, allowing remote control of heating systems to optimize energy use before the start of a journey[12]. These advances in thermal management systems make them increasingly complex, requiring ongoing education for maintenance and repair[12].
The integration of these advanced thermal management systems not only supports the vehicle’s operational efficiency but also aligns with passenger comfort requirements by regulating interior temperature, further underscoring the complexity and importance of these systems in hybrid electric vehicles[4][12].
Environmental and Economic Impacts
Hybrid electric vehicles (HEVs) offer significant environmental benefits by increasing fuel efficiency and reducing pollution levels compared to traditional internal combustion engine vehicles. These vehicles utilize a combination of a battery-powered electric motor and a conventional gasoline engine, which optimizes energy use, especially in urban traffic conditions characterized by frequent stops and starts. This dual system allows hybrids to achieve substantial fuel savings, with some algorithms achieving over 30% savings under certain conditions by minimizing unnecessary fuel consumption during journeys[2][19].
From an environmental perspective, HEVs help mitigate two major challenges faced by conventional transportation: rising oil costs and increasing carbon emissions. Unlike conventional vehicles, hybrids and electric vehicles do not solely rely on oil, and they produce fewer greenhouse gases, making them an attractive option for reducing environmental impact[7].
Economically, hybrids can be more cost-effective in the long run despite potentially higher initial costs. The reduced gasoline consumption in hybrid vehicles compared to their purely gasoline-powered counterparts can result in significant savings over time. Additionally, there are various incentives, such as tax credits for both all-electric and plug-in hybrid vehicles, which can further enhance their economic appeal. These incentives are available at federal and state levels, helping consumers save money and offset the higher purchase prices of hybrids[3][15][20].
Challenges and Future Trends
Hybrid electric vehicles (HEVs) are at the forefront of the transition towards more sustainable transportation solutions, yet they face several challenges that need to be addressed for wider adoption. One of the primary hurdles is the integration of electric vehicles into existing transportation infrastructures, which includes addressing range anxiety, high costs, and the limited availability of charging infrastructure[7]. Public acceptance and awareness of the benefits of EVs remain crucial, as overcoming these challenges involves collaborative efforts among stakeholders, including urban planners and policymakers[7].
The development of HEVs also demands advancements in automotive technologies, particularly in the realms of energy conversion efficiency, driveline consistency, and thermal management[14]. The air-conditioning systems in HEVs play a significant role in cooling batteries and electronics, and their maintenance is becoming increasingly important[12]. Managing the waste heat produced by combined cooling, heat, and power (CCHP) systems is essential to enhance vehicle efficiency and mileage[13].
In terms of future trends, the electrification of vehicles continues to evolve with improvements in battery technology and hybrid systems[13]. The progression towards advanced Hybrid/EV vehicles emphasizes not only higher efficiency but also innovations in cooling system design and thermal management[14]. The hybrid concept, which combines an internal combustion engine with electric vehicle elements, has demonstrated advantages in fuel efficiency and reduced pollution[19]. As technology advances, the importance of specially designed insulated tools for working on high-voltage electrical circuits in hybrid vehicles cannot be overstated[20].
References
[1] U.S. Department of Energy. (2004). How do hybrid electric cars work? Alternative Fuels Data Center. Retrieved December 4, 2024, from https://afdc.energy.gov/vehicles/how-do-hybrid-electric-cars-work
[2] Ramírez, M. (2024, October 12). Evolutionary algorithms in hybrid cars: Optimizing fuel economy. Green Renewables. Retrieved December 4, 2024, from https://en.renovablesverdes.com/hybrid-cars-can-save-fuel-thanks-to-evolutionary-algorithms/
[3] Bonifaccino, M. (2022, June 14). Thermal management in electric and hybrid vehicles. AC Diagnosis. Retrieved December 4, 2024, from https://www.acdiagnosis.com/post/thermal-management-in-electric-and-hybrid-vehicles
[4] Barry, K., Knizek, A., & Shenhar, G. (2024, November 13). How do hybrid cars work? Consumer Reports. Retrieved December 4, 2024, from https://www.consumerreports.org/cars/hybrids-evs/how-do-hybrid-cars-work-a1034181509/
[5] Karataş, İ., Halıcıoğlu, R., & Fedai, Y. (2017). Investigation of hybrid electric vehicle control systems. International Advanced Researches and Engineering Journal, 1(1), 1–6. Retrieved from https://www.researchgate.net/publication/322820362_Investigation_of_hybrid_electric_vehicle_control_systems
[6] APJ Abdul Kalam Technological University. (2019). Control system for electric and hybrid electric vehicles. Studocu. Retrieved December 4, 2024, from https://www.studocu.com/in/document/apj-abdul-kalam-technological-university/electric-and-hybrid-vehicles/control-system-for-electric-and-hybrid-electric-vehicles/22238756
[7] Alanazi, F. (2023). Electric vehicles: Benefits, challenges, and potential solutions for widespread adaptation. Applied Sciences, 13(10), 6016. https://doi.org/10.3390/app13106016
[8] Mirabilis Design. (2020, September 23). Power management of hybrid and electric vehicles. Retrieved December 4, 2024, from https://www.mirabilisdesign.com/power-management-of-hybrid-and-electric-vehicles/
[9] Mullins, A. (2024, March 15). The batteries used in hybrid cars: What you need to know. ShunAuto. Retrieved December 4, 2024, from https://shunauto.com/article/what-batteries-are-used-in-hybrid-cars
[10] Pulse Energy. (2024, July 15). Different types of batteries used in electric vehicles. Retrieved December 4, 2024, from https://pulseenergy.io/blog/types-of-batteries-used-in-electric-vehicles-exploration
[11] U.S. Department of Energy. (n.d.). Batteries for electric vehicles. Alternative Fuels Data Center. Retrieved December 4, 2024, from https://afdc.energy.gov/vehicles/electric-batteries
[12] Xing, Y., Ma, E. W. M., Tsui, K. L., & Pecht, M. (2011). Battery management systems in electric and hybrid vehicles. Energies, 4(11), 1840–1857. https://doi.org/10.3390/en4111840
[13] U.S. Department of Energy. (2024). Thermal management in electric and hybrid vehicles. HELLA Tech World. Retrieved December 4, 2024, from https://www.hella.com/techworld/us/Technical/Car-air-conditioning/Thermal-management-in-electric-and-hybrid-vehicles-1725/
[14] Brainly. (2022, October 31). What is used on some electric and hybrid vehicles to cool the power electronics and charger modules? Brainly. Retrieved December 4, 2024, from https://brainly.com/question/29215604
[15] RIGID HVAC. (2024, April 10). What types of battery cooling systems are used in EVs and HEVs? Retrieved December 4, 2024, from https://www.rigidhvac.com/blog/types-battery-cooling-systems-for-evs-hevs
[16] Huang, J., Shoai Naini, S., Miller, R., Rizzo, D., Sebeck, K., & Shurin, S. (2019). A hybrid electric vehicle motor cooling system—Design, model, and control. IEEE Transactions on Vehicular Technology, 68(5), 4113–4122. https://doi.org/10.1109/TVT.2019.2902135
[17] Kurz, J. (2000, October 1). Hybrid electric vehicles: A history of technological innovation. Illumin. Retrieved December 4, 2024, from https://illumin.usc.edu/hybrid-electric-vehicles-a-history-of-technological-innovation/
[18] U.S. Environmental Protection Agency. (2023, October 1). Electric & plug-in hybrid electric vehicles. Retrieved December 4, 2024, from https://www.epa.gov/greenvehicles/electric-plug-hybrid-electric-vehicles
[19]Tung, S. C., Woydt, M., & Shah, R. (2020). Global insights on future trends of hybrid/EV driveline lubrication and thermal management. Frontiers in Mechanical Engineering, 6, Article 571786. https://doi.org/10.3389/fmech.2020.571786
[20] Brainly. (2024, February 11). When working on the ____circuits of a hybrid vehicle, a technician should only use specifically designed insulated tools (rated at 1000 volts) [Answer]. Brainly. Retrieved December 4, 2024, from https://brainly.com/question/47539822
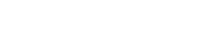